Key Facts
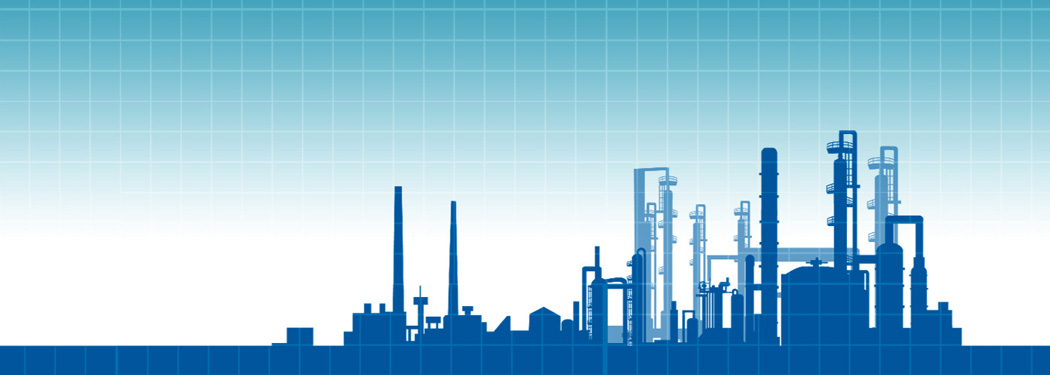
Introduction
-
Oil and gas refining is an inherently energy-intensive activity. Refineries require energy, in the form of heat and motive power, to transform crude oil or natural gas into commercial products.
-
Like other energy-intensive industries (EIIs), the sector is under pressure to reduce emissions sharply to help limit global warming and meet climate targets. The EU has committed to reduce at least 40% of its greenhouse gas (GHG) emissions by 2030, against 1990 levels and to become climate neutral by 2050.
-
Direct emissions from oil refining typically range from 100 to 200 kg CO2/tonne crude oil. The carbon intensity varies for each refinery and depends on the complexity, energy efficiency and ratio of feedstocks.
-
Carbon capture and storage (CCS) is needed for carbon-intensive industries because fuel switching is often not an option, or process-related emissions cannot be avoided.
-
CCS cost is identified as the most significant hurdle limiting its industrial uptake in the short-to-medium term. REALISE aims to enable a decrease of at least 30% in CO2 capture costs compared with current benchmarks in industrial clusters around oil refining.
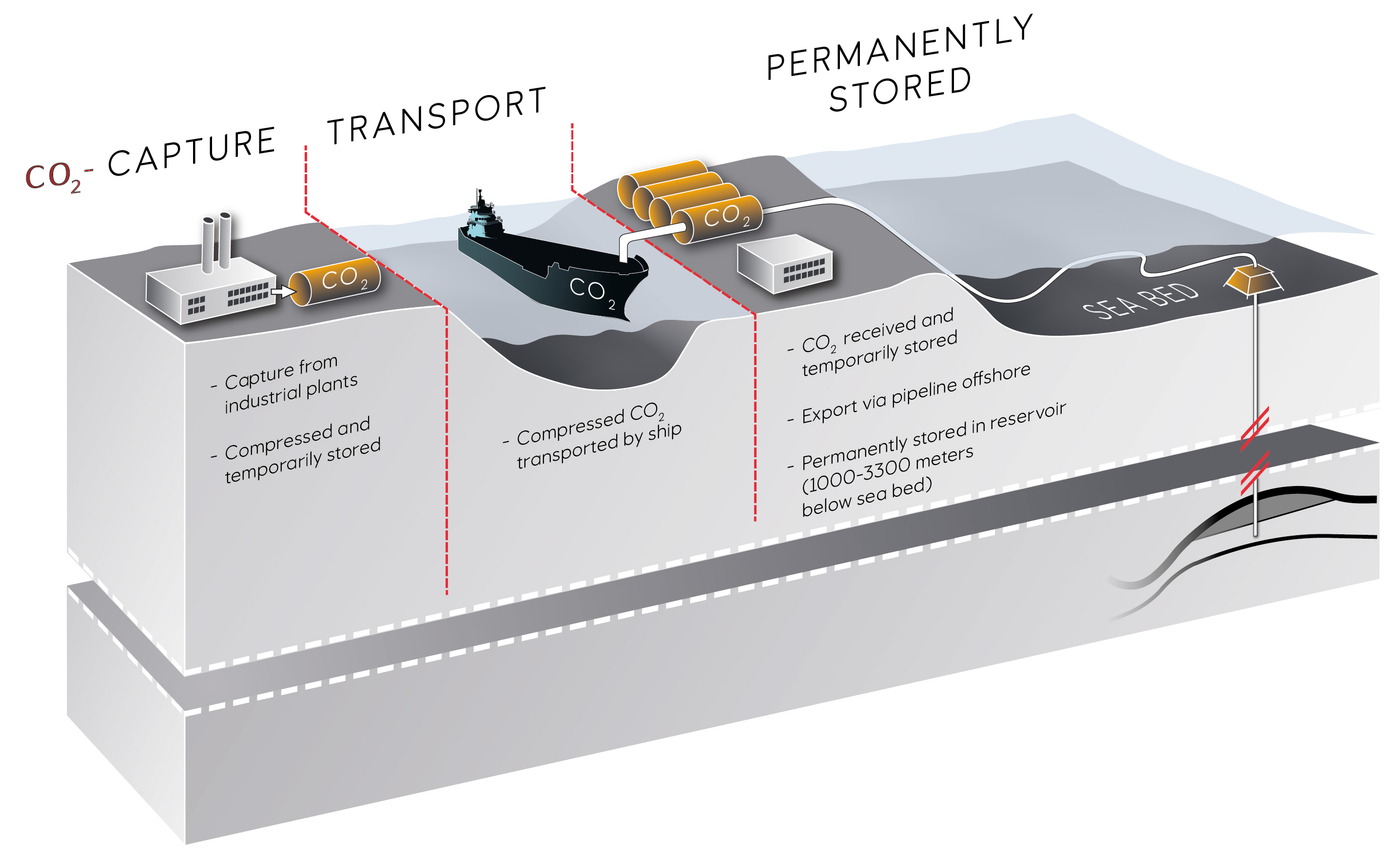
CCUS and refineries
-
The refining sector contributes about 4% of the global CO2 emissions associated with human activity, the third highest among stationary CO2 producers after power and cement.
-
Emissions come from a large number of relatively small sources. A large number of stacks emit flue gases of varying CO2 concentration and sulphur content. This pushes up costs and contributes to relatively low capture rates. Each refinery is different so CO2 capture systems must be individually tailored.
-
At present, the maximum potential CO2 that can be captured across a refining site is limited to about 70% of CO2 available. REALISE aims to increase this potential to 90%.
-
In a typical complex refinery, the main emissions come from: power generation (24% of total); fluid catalytic cracking (FCC), a crude oil conversion process (13%); steam methane reforming (SMR), the main method for producing hydrogen (12%); and the distillation units (20%).
-
The European refinery sector’s total 2050 emissions would be reduced by 70% with the deployment of CCS, compared to 50% with no CCS, according to Concawe.
-
Electrification across sectors, such as transport and heating, is set to push down demand for fuel. Concawe studies assume a reduction in total demand of 13% in 2030 versus 2014, and from 20–35% by 2050 versus 2030.
-
However, there will still be significant demand for liquid fuel – and thus for CCS – because electrification is not yet fully possible in many segments, including commercial transport, aviation, shipping and petrochemicals.
-
There have been relatively few CCUS projects involving refineries. Notable examples are Japan’s Tomakomai project and Sinopec’s Zhongyuan project in China’s Henan province, both large-scale demonstration projects.
-
At Tomakomai, CO2 was captured from a hydrogen production unit at a refinery and injected offshore under the seabed. The Sinopec project is the world’s largest CCUS facility at a fluid catalytic cracking operation. Once liquefied, the captured CO2 is transported by truck to a nearby oil field and used in enhanced oil recovery (EOR).
-
ReCAP, funded by the Norwegian government, the IEA and Concawe and run by SINTEF, the lead partner in REALISE, simulated retrofitting CO2 capture in four oil refinery base cases, selected as representative of the European sector. It focused on post-combustion capture with monoethanolamine (MEA) solvent, a chemical absorption process, a technology REALISE will also employ.
-
CO2 capture from cracker gas (RFCC) has also been demonstrated at the test facility at Norway’s Technology Centre Mongstad (TCM), a member of REALISE’s Expert Advisory Board, also using 30 wt% MEA.
-
REALISE will build on these projects’ findings, further developing the technologies examined and lowering the costs of CO2 capture by at least 30% against current benchmarks. ReCAP estimated the cost of retrofitting capture technology at between 160 and 210 $ per tonne of CO2 avoided. Separate research from Concawe put costs at 80-180 € per tonne depending on source, scale and technology.
Uses for captured CO2
A glass of champagne contains about a million bubbles of CO2 created naturally during fermentation. In soft or alcoholic drinks, such as cola, cider and beer, it is added to make them carbonated or fizzy. This is one of the uses for captured CO2, but utilisation options remain underdeveloped. Below are some of the present uses of CO2, as well as areas of potential breakthrough in the next decade.
-
Food and drink: A typical carbonated soft drink includes purified water that has been impregnated with CO2 gas. The dissolved gas not only adds a distinctive taste and sparkle but also acts against bacteria. CO2 plays a vital role in extending the shelf life of fresh meat and salads. It is also used in the bottling and kegging process for beer.
-
Enhanced oil recovery (EOR): A well-established technology, EOR is the biggest industrial use for CO2, accounting for about 90% of the total, mainly in the US. It involves injecting CO2 into existing oil reservoirs to boost recovery rates. Around 20% of global oil production comes from EOR. Most of the CO2 used comes from natural underground sources; the challenge is to boost the proportion coming from carbon capture.
-
Concrete: new processes could lock up CO2 permanently in concrete. Precast structural concrete slabs could be made with new types of cement that, when cured in a CO2-rich environment, produce concrete that is around 25% CO2 by weight. A second concrete process involves synthetic CO2-absorbing aggregates (combining industrial waste and carbon curing). This can produce concrete which is more than 40% CO2 by weight.
-
Fuel: Captured CO2 can be combined with hydrogen to create synthetic petrol, jet fuel and diesel. The key is to produce the hydrogen sustainably. The aviation industry is especially keen to see development of synthetics fuels: it consumes a lot of energy and its emissions are difficult to abate.
-
Plastics: CO2 can be used instead of fossil fuel-based inputs in plastics production. The chemicals industry is testing a range of CO2-based plastics for widespread use, given increasing demand from environmentally aware consumers. Green polyurethane, for example, used in textiles, flooring for sports centres and mattresses, is in the early stages of commercial roll-out.
-
Biochar: Created by heating farm biomass waste in an oxygen-poor environment, biochar is added to soil to bring certain benefits, such as the potential to increase crop yields by 10%. Producing it captures about half of the CO2 that would otherwise escape during waste decomposition.
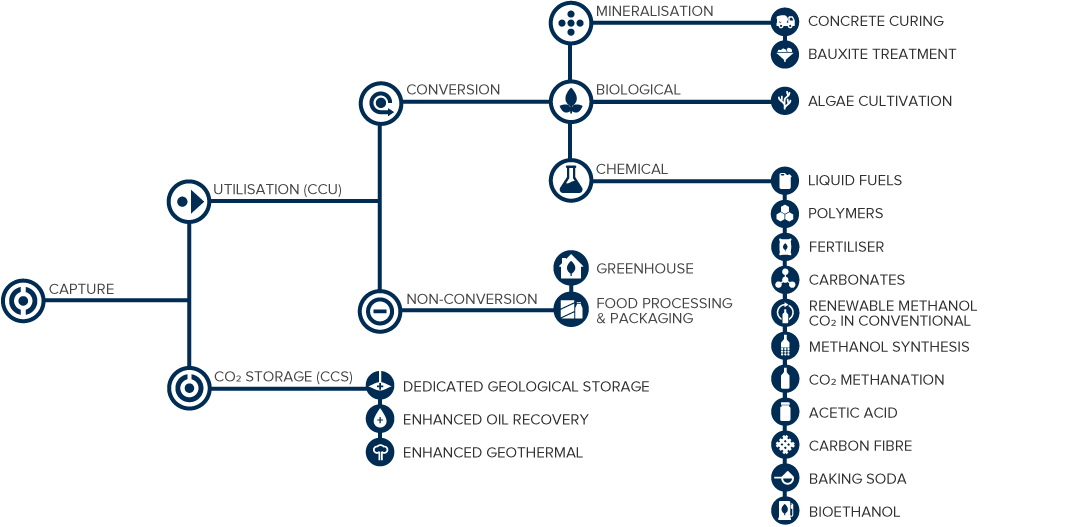
CO2 storage
Capturing CO2 only makes sense if it can be either reused or safely stored for the long term. With CO2 reuse limited, the majority of captured CO2 will be stored deep underground in geological formations.
-
The world's first offshore CO2 storage, in the North Sea, has been operated by Equinor, a partner in REALISE, since 1996. Nearly one million tonnes of CO2 is stored annually in the Utsira formation with an additional 700,000 tonnes a year stored in a subsea formation in the Snøhvit field since 2008.
-
There does not appear to be a shortage of suitable formations. On some estimates, the US, for example, could geologically store 500 years of its current rate of CO2 emissions. Globally, the number is around 300 years. In western Europe, there are promising storage structures in the North Sea.
-
Rather, the barriers to storage are non-technical such as the legal complexities over, for example, underground property ownership or liability for potential leaks. Public support for CCS must also be built.
-
A further issue is CO2 transportation, presently a weak link in the value chain. In the US, there are only around 5,000 miles of pipeline transporting CO2, compared with 300,000 natural gas pipelines . Outside the US, CO2 pipelines are rare. Reasonably easy transport to suitable storage is thus a key condition for CO2 capture to be a realistic proposition for refineries. This can involve pipelines or shipping or a combination of both.
-
REALISE will assess the CCUS chain, from emitters to permanent and safe storage. We will draw on the expertise of our partners Equinor and Ervia, who are developing CCUS in the promising Northern Lights and Cork industrial cluster projects respectively.
-
REALISE will develop full CCUS chain integration strategies for three business cases, including in Ireland and China. European refineries can benefit from the CO2 transport and storage opportunities presented by the EU’s Projects of Common Interest (PCIs) which support cross-border energy infrastructure. Both the Northern Lights and Cork projects are PCIs which benefit from a simplified permitting process and access to EU funding from the Connecting Europe Facility. Linking refineries to PCIs would lower risk and increase their chances of developing viable CCUS schemes.
-
REALISE will work to increase public awareness of and support for CCUS through education and engagement programmes. While the technology’s role in reducing emissions is welcomed, past experience shows there can be resistance to deployment of CCUS in specific locations. To help measure attitudes, REALISE will develop a societal readiness index as part of the three business cases.
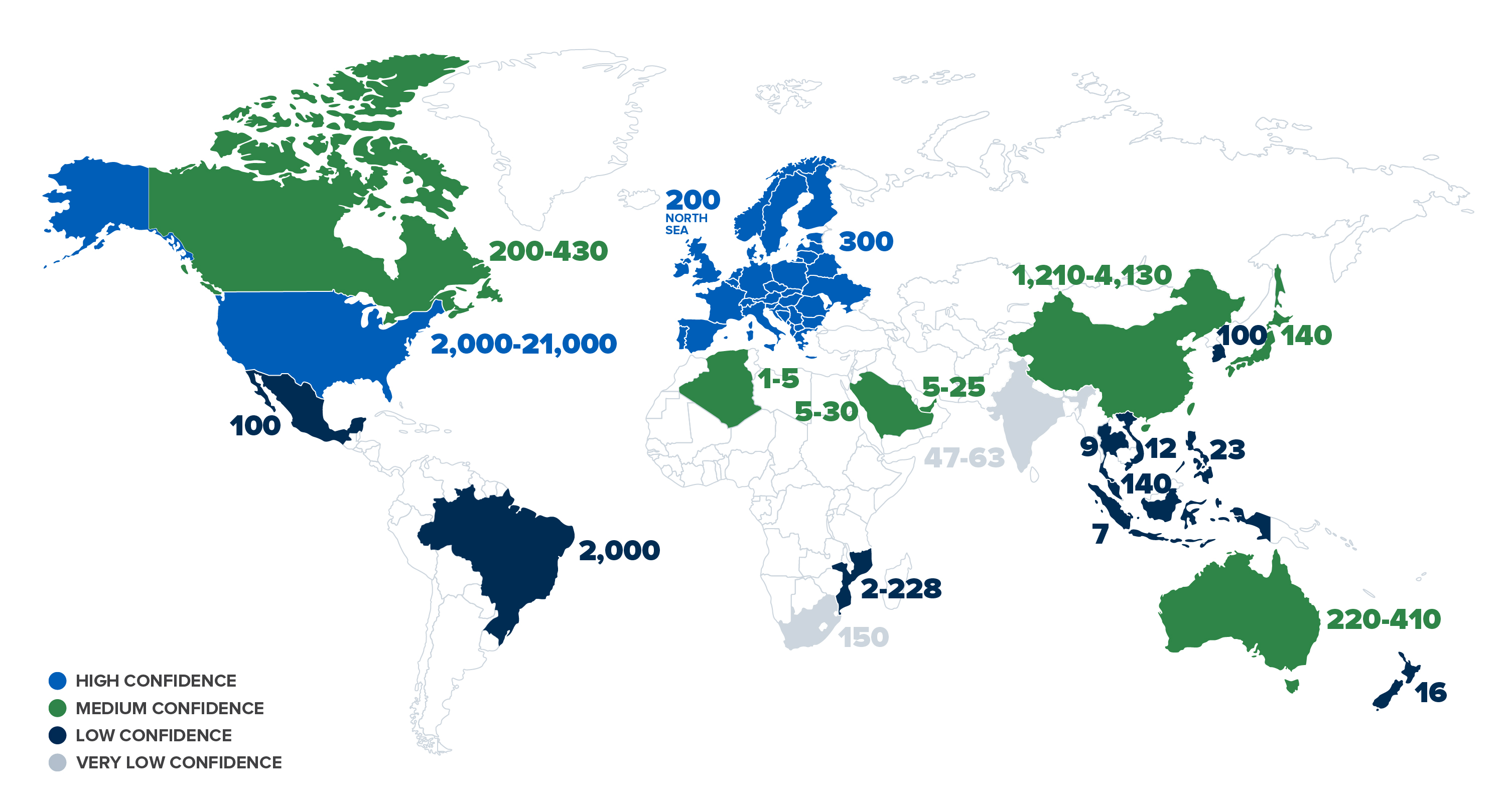